Remote Synchronization in Stuart-Landau Oscillators
This work was done along with Prof. Michael Rosenblum. Our submitted manuscript: click here
Aim -
To explain the phenomenon of 'remote synchronization' using a simple motif of three Stuart-Landau oscillators in a chain, using numerical and theoretical techniques.
Summary -
Synchronization is a ubiquitous phenomenon observed in man-made and natural systems alike, ranging from a spectacular synchronous display by fireflies in the forests of Thailand to the uncanny but satisfying behavior of metronomes in lockstep when placed on a wobbly platform. These examples are shown in the following videos. In general, any object with an inherent oscillatory characteristic (e.g., pendulum clocks) may synchronize. In the technical jargon, oscillatory objects are (unsurprisingly) referred to as 'oscillators,' and each oscillator has its characteristic speed of oscillating called the 'natural frequency.' Given its pervasiveness, synchronization has received a lot of attention from physicists, applied mathematicians, biologists, and engineers. While certain aspects of synchronization are well-understood, yet others pose significant challenges.
Vid 1a: Synchronizing fireflies in the forests of Thailand. The synchronization is most spectacular around 37s into the video
Vid 1b: Synchronization between 5 metronomes
To understand the outcomes of this work, we must first discuss an interesting study by Bergner et al. (2012). They considered a star network of Stuart-Landau oscillators with one hub and several other peripheral oscillators, as shown in Figure 1. (The Stuart-Landau oscillator is a paradigmatic mathematical model of an oscillator.) Moreover, the peripheral oscillators have similar natural frequencies, while the hub is significantly mismatched. This simple network represents a feature common in real-life networks; for example, on a social media platform (e.g., Twitter), thousands of common people (similar peripheral nodes) are 'connected' to a celebrity (mismatched hub). Bergner et al. observed that the peripheral oscillators synchronize readily in such networks, despite the absence of direct connections between them. This phenomenon is known as 'remote synchronization.' Since then, remote synchrony has been observed in more complex and real-life networks. It is quite plausible that this phenomenon may govern crucial dynamics in the real world; the coherent functioning of distant regions in the brain despite any direct connections between them may have its root in remote synchrony.
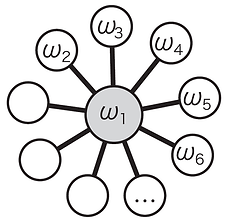
Fig 1: A star network. Oscillator 1 is the hub while the others are peripheral oscillators. 'omega' denotes the natural frequency. (taken from Bergner et al. (2012))
Separate (earlier) studies discerned two key factors responsible for inducing remote synchronization. However, a simultaneous consideration of both factors along with a comprehensive theoretical understanding was still lacking, until this work. As part of this study, we considered the simplest possible star network consisting of a single hub and two peripheral nodes. We further simplified each oscillator to its essential component alone, namely, its phase. With the phase equations, we derived analytical expressions that explained when and why a system exhibits remote synchronization. These equations elucidated a hitherto unreported subtle interplay between the two key factors earlier reported in the literature. Finally, the analytical predictions were successfully validated against their numerical counterparts.
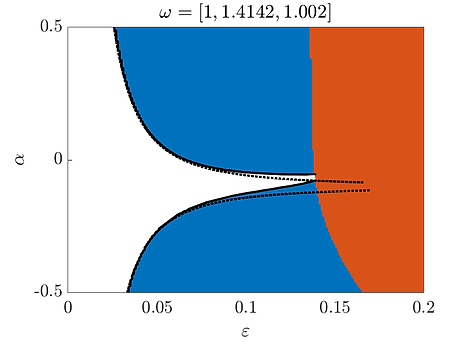
Fig 2: The transition to remote synchrony predicted by our theoretical investigations against the numerical results on the coupling strength (ε) versus non-isochronicity (α) plane. The solid blue region (â– ) shows the actual region of remote synchrony (computed numerically without any approximations). The solid black line (――) is the border of this region computed numerically after applying the second-order phase reduction. The dashed black line (- - -) is the same border, but calculated analytically, with further approximations. The match between all three methods is perfect for small coupling strengths, thereby validating our methodology.
What I learnt -
-
Numerical simulations concerning synchronization (on MATLAB)
-
Application of perturbation methods
Current Status -
Manuscript submitted for publication
Rough Timeline -
1st June 2021 - 31st July 2021
References -
-
Vid 1a: robin meier, Synchronizing Fireflies, YouTube (accessed July 2021)
-
Vid 1b: uclaphysicsvideo, spontaneous synchronization, YouTube (accessed July 2021)
-
Bergner, Andre, et al. "Remote synchronization in star networks." Physical Review E 85.2 (2012): 026208.
-
Vlasov, Vladimir, and Angelo Bifone. "Hub-driven remote synchronization in brain networks." Scientific reports 7.1 (2017): 1-11.
-
Gambuzza, Lucia Valentina, et al. "Analysis of remote synchronization in complex networks." Chaos: An Interdisciplinary Journal of Nonlinear Science 23.4 (2013): 043103.
-
Gengel, Erik, et al. "High-order phase reduction for coupled oscillators." Journal of Physics: Complexity 2.1 (2020): 015005.
-
Pikovsky, Arkady, et al. Synchronization: a universal concept in nonlinear sciences. No. 12. Cambridge university press, 2003.